Hydrogen peroxide serves as a precursor of the hydroxyl radical
Triplet oxygen initially acquires one electron to form the superoxide anion radical. Subsequently, it gains another electron to become peroxide, to which two protons combined to form hydrogen peroxide. We examined those processes in detail in the previous article.
From the perspective of hydrogen peroxide, it starts with 6 outermost electrons and acquires one electron at a time to have 8 electrons, satisfying the octet rule. All electrons are also paired, so it can no longer be called a radical. However, this hydrogen peroxide is quite important in that it can create the hydroxyl radical, which is the most notorious of radical of all. Fortunately, our human body has enzymes capable of intercepting hydrogen peroxide before it generates hydroxyl radicals. We have two such enzymes. However, unfortunately, there is no way to directly deal with and neutralize the hydroxyl radical, which can cause the greatest harm, so blocking it in advance of its birth can be said to be an essential self-rescue measure. It's like preventing it by nipping it in the bud. (The Terminator suddenly comes to mind...)
Fenton reaction and Haber and Weiss reaction
There are several reactions that produce hydroxyl radicals. First, let's look at the most well-known reaction that generates hydroxyl radicals, called the Fenton reaction. Hydrogen peroxide has a structure with two oxygen atoms in the center and a hydrogen atom bonded to each side. It has a tendency to easily break these bonds when exposed to external stimuli such as heat. The cleavage occurs symmetrically, dividing the molecule into two equal halves. This process is known as homolytic cleavage. As a result, the covalent bonds that held the shared electrons are broken, releasing one electron each, and forming two unpaired radicals. The eight outermost electrons are now distributed as seven each, resulting in two radicals with unpaired electrons.
This clearly illustrates the distinction between ions and radicals. Ions achieve stability by either gaining or losing electrons to complete their outer electron shell, while radicals remain unstable because they possess unpaired electrons. Let's take a closer look at the process described above.
One electron is obtained from the surrounding ferrous transition metal iron (Fe2+). The radical that acquires this electron forms a stable hydroxide ion by pairing with it, while the remaining radical becomes a hydroxyl radical. Copper, another transition metal with a 2+ oxidation state, can also play a catalytic role similar to iron. In our bodies, hydrogen peroxide reacts with iron to produce hydroxyl radicals, a process known as the Fenton reaction. Additionally, during this reaction, iron, which loses electrons and becomes Fe3+ cation, can combine with superoxide radicals and be reduced (gain electrons) back to its original Fe2+ state. The overall combination of these two reactions is referred to as the Haber-Weiss reaction, and can be expressed as follows.
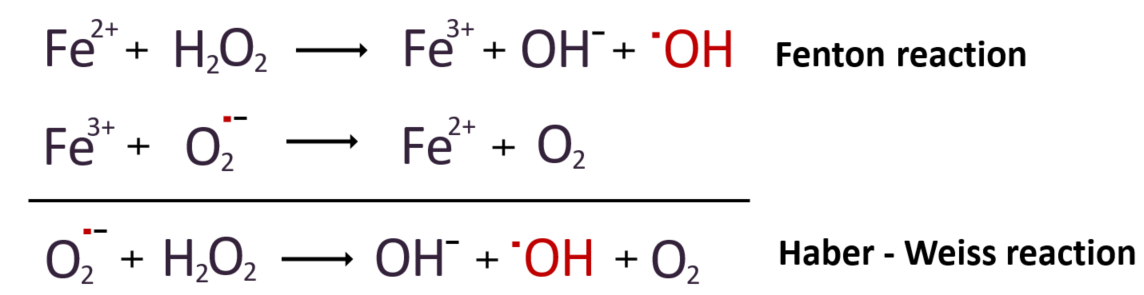
Since iron ions and superoxide anion radicals can both exist in our blood, it means that hydroxyl radicals can be generated within the human body. Hydroxyl radicals can also be produced by irradiating water with gamma rays. During daylight hours, some of the oxygen atoms formed by the photolysis of ozone due to solar radiation react with water vapor, resulting in the generation of hydroxyl radicals. These hydroxyl radicals formed in this way are crucial for cleansing the atmosphere by oxidizing pollutants. However, most of them are eliminated through reactions with carbon monoxide and methane in the troposphere.
Hydroxyl radicals have gained a notorious reputation for their high reactivity and ability to react rapidly with surrounding substances. Even within our bodies, they can readily and quickly react with biomolecules such as proteins, lipids, and nucleic acids. These interactions can lead to the destruction of cell membranes, alterations in protein structure and function, and mutations in DNA. If such damage persists over a long period of time, it can contribute to cellular dysfunction and give rise to various diseases, including aging and cancer. It undermines the integrity of surrounding cells, just like getting a person soaked completely up to the underwear in drizzling rain.
At this point, let's look at the lipid peroxidation process as a specific example of how hydroxyl radicals play a dangerous role in the human body.
Lipid Peroxidation
A good demonstration of the danger posed by hydroxyl radicals is lipid peroxidation. In the first article related to reactive oxygen species, I briefly mentioned the alarming chain reaction of radicals in the previous article. Let's dig into the mechanism by which hydroxyl radicals encounter lipids, triggering an unstoppable chain reaction with devastating outcomes.
One of the important parts of the human body composed of lipids is the cell membrane, including the cell membrane that surrounds the cell itself and the one surrounding various organelles within the cell. Membranes consist of a double layer(bilayer) of phospholipids. These phospholipids consist of a so-called “head part” made of phosphate and a “tail part” made of fatty acids. The head part is hydrophilic, meaning it likes water, whereas the fatty acids are hydrophobic, meaning they repel water. Consequently, the head part faces outward, while the fatty acids are nestled inward, avoiding contact with the cellular moisture as much as possible. I found the most visually representative image on the internet (see the image below).
Polyunsaturated fatty acids (PUFAs), including omega-3 and omega-6 fatty acids, are also part of these fatty acids. The double bonds in PUFAs cause the fatty acid to bend, allowing the phospholipids to be more flexible and fluid. However, the issue lies in the methylene bridge (-CH2) located between double bonds of polyunsaturated fatty acids. This methylene bridge contains two highly reactive hydrogen molecules, which can make PUFA a vulnerable target of the radicals to steal electrons. As a result, the polyunsaturated fatty acid lipid is transformed into a radical.
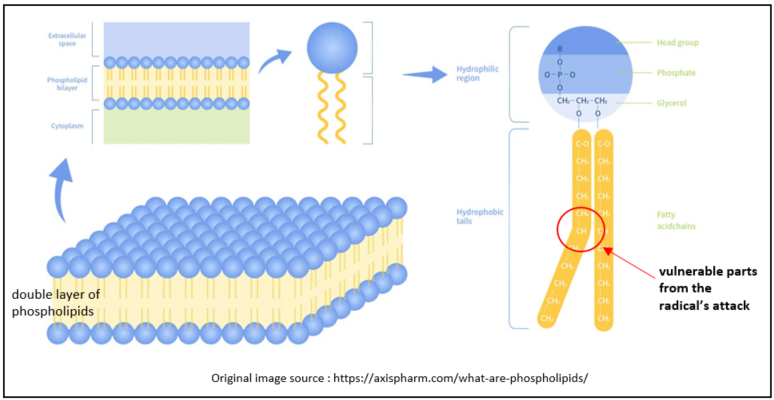
Lipid peroxidation goes through three stages: initiation, propagation, and termination.
1. Initiation
Hydroxyl radicals attack polyunsaturated fatty acids (LH), which are components of cell membrane lipids, by stealing hydrogen atoms to form water and converting unsaturated fatty acids into lipid radicals.

Then, the lipid radicals react with nearby oxygen to form another radical called lipid peroxyl radical.

2. Propagation
The lipid peroxyl radical, in turn, attacks other nearby lipids (LH) to form lipid hydroperoxide(LOOH) and converts those lipids into lipid radicals.

The lipid radicals formed in this way continue to initiate the process and propagate radicalization to surrounding lipids.

Furthermore, the lipid hydroperoxide(LOOH) generated in this process can aggravate the lipid peroxidation chain reaction by reacting with nearby iron ions (Fe2+ or Fe3+) to produce alkoxyl radicals, lipid molecules that are bonded to oxygen. These alkoxyl radicals can then attack unsaturated fatty acids in the cell membrane, further worsening the lipid peroxidation chain reaction.
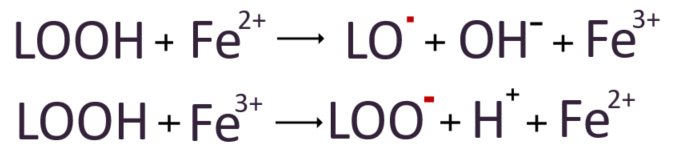
3. Termination
When the concentration of radicals increases excessively due to the repeated chain reaction, it eventually reaches a point where the radicals start reacting with each other. This termination of the chain reaction occurs as radicals combine to form non-radical molecules. Although no more radicals are produced once the chain reaction stops, the numerous lipid peroxides generated in this process still pose a risk of damaging lipid membranes. The extent of this damage can range from a reduction in cell membrane fluidity to a complete breakdown of the integrity of the phospholipid bilayer.
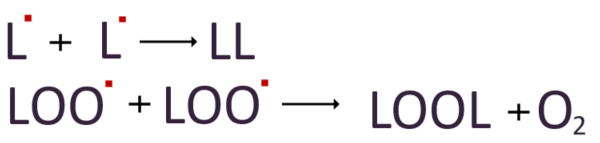
The problem is that these lipid peroxides react with metal ions such as iron and copper and are converted into highly reactive aldehydes. These aldehydes react with proteins, nucleic acids, and glutathione (GSH) to form dangerous adducts such as malondialdehyde(MDA) or 4 -hydroxy-2-nonenal(4-HNE). is to form. These toxic aldehydes, which are the final products of lipid peroxidation, are known to induce cholesterol oxidation, leading to atherosclerosis and cardiovascular diseases. MDA is also used as a biomarker to measure the progress of lipid peroxidation by assessing its concentration.
The role of antioxidant enzymes and antioxidant substances
Do our bodies have mechanisms to countermeasure lipid peroxidation caused by the attack of hydroxyl radicals? Fortunately, yes. Through the activity of various antioxidant enzymes, superoxide radicals, which can be considered as the ancestors of hydroxyl radicals, can be firstly converted into hydrogen peroxide and then transformed into harmless water, effectively preventing the generation of hydroxyl radicals. Additionally, in cases where lipid peroxidation occurs as a result of radical chain reactions, there are antioxidant substances that willingly sacrifice themselves to intercept and inhibit this process. In the following articles, we will explore the role of antioxidant enzymes and antioxidant substances in our body's defense system against oxidative stress caused by these radicals.