In the human body, the cascade of reactive oxygen species initiates with superoxide radicals, which then progress to peroxides, and ultimately result in hydrogen peroxide. This process can be seen as a gradual weakening of the oxidative intensity. While the human body does not have a direct means to control the most lethal hydroxyl radical, it does have a mechanism to deal with it as a preemptive measure by converting hydrogen peroxide, a precursor and medium of hydroxyl radical, into harmless water. Let's explore step by step the activities of the enzymes involved by examining how the human body processes these reactive oxygen species, along the chain of active oxygen.
Under physiological conditions, hydrogen peroxide maintains relative stability and interacts with a variety of biologically important compounds. It is recognized for its relatively low toxicity due to its slower reaction speed with biomolecules. The primary mechanism for eliminating reactive radicals mainly relies on hydrogen peroxide, taking advantage of its inherent characteristic of easy decomposition. Superoxide radicals, the starting point of reactive oxygen cascades, are also converted to hydrogen peroxide before proceeding to the next step.
Step 1: Convert superoxide radicals to hydrogen peroxide.
Superoxide dismutase (SOD)
Superoxide dismutase(SOD) is an enzyme that facilitates the conversion of superoxide radicals into hydrogen peroxide. The name itself explains its function: "dismutase" denotes dismutation, with the suffix "-ase" indicating an enzyme. Dismutation refers to a chemical reaction in which one substance undergoes simultaneous oxidation and reduction, yielding different products. While this reaction occurs naturally at a slow pace in nature, its speed can be significantly enhanced through the use of a catalyst. In the case of SOD, two superoxide radicals and two protons undergo oxidation and reduction reactions simultaneously, resulting in the production of hydrogen peroxide and oxygen. SOD serves as the catalyst that speeds up this reaction.
SOD Family
The SOD family consists of three isoforms: SOD1, SOD2, and SOD3. An isoform refers to proteins or enzymes that are derived from the same gene but have slightly different structures or functions. SOD1 is predominantly located in the cytoplasm, SOD2 is mostly found within mitochondria, and SOD3 is present extracellularly, often in locations such as cerebrospinal fluid and blood vessels in the brain. SOD has a large and complex protein structure and contains metal ions in their active sites, which play a crucial role in their catalytic activity. SOD1 and SOD3 utilize copper and zinc ions, respectively, while SOD2 utilizes manganese ions at the center of their catalytic reactions. These metal ions assist in the movement of electrons and facilitate the catalytic functions of SOD enzymes.
Role of metal ions (copper, zinc, and manganese)
Let's briefly examine the mechanism of SOD1 to understand the role of metal ions in this reaction. Copper ions are crucial components of SOD1. [1]
In the first step, one superoxide radical molecule donates an electron to a copper ion (oxidation) and becomes an oxygen molecule. Simultaneously, the Cu2+ ion gains an electron (reduction), leading to its conversion into Cu. During this process, the bond between the histidine residue of the active site, which binds to Cu, and the Cu ion is broken, and His63 Nε1 (meaning the nitrogen at the first position in the side chain of histidine at position 63) is protonated. Protonation means the attachment of a hydrogen cation(proton) to an atom, molecule, or ion.
In the second step, the proton received from His63 Nε1 and one electron from Cu are donated to the superoxide radical. Cu, in turn, donates an electron and undergoes oxidation to revert to Cu2+, while the superoxide radical gains one electron and undergoes reduction. As a result, the broken bridging bond with histidine is restored to its original state. The role of metal ions is crucial in this process. This implies that in order to properly remove radicals from our body, it is important to consume minerals such as copper, zinc, and manganese appropriately.
SOD converted the superoxide radical into hydrogen peroxide. However, it cannot be considered a completely satisfactory safety mechanism. Therefore, in the next step, let's look at two majoy enzymes involved in the detoxification of hydrogen peroxide.
Step 2: Convert hydrogen peroxide to water.
As we saw in the previous article, hydrogen peroxide is produced from super radical anions.

Additionally, as seen above, super radicals are catalyzed by SOD to form hydrogen peroxide. Our body first converts radicals into hydrogen peroxide and then converts them into harmless water to neutralize the effects of radicals. There are several antioxidant enzymes involved in this detoxification process. However, the enzyme known to be the most powerful and fastest-acting among these is Glutathione Peroxidase, or GPx.
1. Glutathione Peroxidase (Gpx)
GPx enzyme is an enzyme that rapidly converts radicals into water, and selenium and glutathione are essential for the action of this enzyme. Glutathione is a powerful antioxidant that we are already familiar with, consisting of three amino acids: glutamate, cysteine, and glycine. GPx is distributed in various organs of the body, including the cytoplasm, mitochondria, and peroxisomes, found in organs such as the liver, red blood cells, kidneys, and brain.
The active site of GPx contains selenocysteine (Sec), which is crucial for its catalytic activity. Selenocysteine has the same structure as Cysteine amino acid, and the difference is that selenium atom takes the place of sulfur. Like Cystein has a thiol functional group(SH) in which a sulfur(S) atom and a hydrogen(H) atom are bonded, Selenocysteine also has a Selenol group(Se-H) with the bond between a selenium(Se) atom and a hydrogen(H) atom.
1. Selenol of the GPx enzyme reacts with the radical first. Se-H (selenol) becomes Se-OH (selenic acid) by receiving an oxygen atom from lipid peroxide (ROOH) or hydrogen peroxide (H₂O₂). At this time, ROOH, which has lost one oxygen atom, is detoxified into alcohol (ROH), and H₂O₂ is detoxified into H₂O.

2. OH of selenic acid (Se-OH) of GPx combines with one hydrogen atom received from GSH to generate H₂O, and these form glutathiolated selenol (Se-SG).

3. Here, GPx is ready to resume enzymatic action again by receiving the hydrogen released by one molecule of GSH and returning it to Se-H. At this time, the two GSH molecules that give up hydrogen in turn form a disulfide bond to become GSSG, that is, oxidized glutathione.

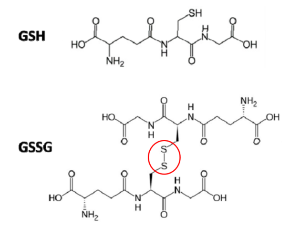
Antioxidant action by GPx
1-1 Glutathione Reductase(GR)
GSH neutralizes the radical, restores the GPx enzyme to its original state, and oxidizes itself to form GSSG, or oxidized glutathione. In order to continuously reactivate GPx, the most powerful antioxidant, we must reduce the oxidized GSSG, restore it to its original state, and reintroduce it to antioxidant activity. Fortunately, the human body possesses an enzyme called Glutathione Reductase (GR) for this purpose. Reduction of GSSG requires an electron donor, which is provided by NADPH. Essentially, the core of the GR catalytic enzyme relies on NADPH, an electron donor generated in the hexose monophosphate (HMP) shunt, an alternative pathway branching off from glycolysis and proceeding in parallel with it. This pathway, also known as the pentose phosphate pathway (PPP), enables the production of NADPH, sustaining the antioxidant activity of glutathione antioxidants. Additionally, the PPP pathway synthesizes ribose-5-phosphate, a component of nucleotides essential for DNA and RNA molecules. Glucose (C6H12O6), a six-carbon sugar, can enter either glycolysis or the alternative HMP shunt pathway, depending on the metabolic demands of the cell at the time.
Glutathione reductase (GR) is a protein that relies on FAD (flavin adenine dinucleotide) for its function. Initially, NADPH donates electrons to FAD, resulting in the reduction of FAD to FADH2. Then, the reduced FADH2 transfers electrons from the active site of GR to GSSG. This process reduces hydrogen protons on two cysteine residues in GSSG, yielding two molecules of GSH and restoring them to their reduced state. Consequently, GR acts as a catalyst, reducing one molecule of GSSG to two molecules of GSH, and plays a crucial role in maintaining the balance of reduced and oxidized GSH within the cell.

For your reference, FAD is derived from riboflavin, a well-known essential vitamin B2, while NAD and NADPH are derived from vitamin B3, niacin. Riboflavin is not synthesized within the body, and although niacin can be produced by converting the amino acid tryptophan, the amount produced may not be sufficient. Therefore, it is important to ensure an adequate intake of these vitamins through food or other means.
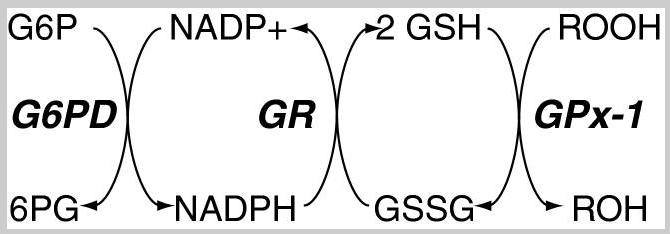
[3] Redox pathways that maintain essential cofactors of Gpx
GSH:GSSG ratio
The above picture summarizes the steps glutathione detoxifies reactive oxygen species (ROS) and the enzymes required for each step. Our bodies utilize these enzymes to continually regenerate antioxidant activity. The ratio of reduced glutathione (GSH) to oxidized glutathione (GSSG) cdetoxifyan serves as an indicator of cellular health [4]. In a normal cellular environment, GSH predominates at a much higher concentration, with a typical ratio of GSH to GSSG around 100:1. However, in situations of oxidative stress, this ratio may decrease significantly, dropping to 10:1 or even 1:1[5]. This ratio serves as a measure of the degree of oxidative stress within cells. Lower levels of GSH may suggest an increase in oxygen radicals, a decrease in antioxidant capacity, or an impairment of glutathione recycling mechanisms. Monitoring this ratio can offer valuable insights into the extent of damage caused by oxidative stress in cells and their susceptibility to related diseases.
2. Catalase
Catalase is an enzyme responsible for detoxifying hydrogen peroxide that exists in high concentrations within the peroxisomes, the intracellular organelles where various reactions generating hydrogen peroxide take place, such as fatty acid decomposition. It makes sense that catalase is observed in large amounts in peroxisomes to maintain the balance of the abundant hydrogen peroxide. Of course, catalase is also found outside the cell.
Various enzymes work to reduce the concentration of hydrogen peroxide, but in cases where the concentration exceeds 1 mM(micromole), catalase becomes the primary defense enzyme[6]. Such concentrations are commonly observed in situations of oxidative stress.
Catalase is a large tetrameric protein made up of four polypeptides with more than 500 amino acids linked together. Each tetramer contains four heme groups as its subunits, which directly react with hydrogen peroxide. The heme group has a porphyrin ring structure with iron(Fe3+) in the center, and this part acts as a coenzyme, facilitating electron transfer between hydrogen peroxide through oxidation and reduction reactions. This process eventually breaks down two molecules of hydrogen peroxide into two molecules of water and one molecule of oxygen. In the image below, the four orange dots represent the heme groups, which are the active sites for catalase catalytic action.
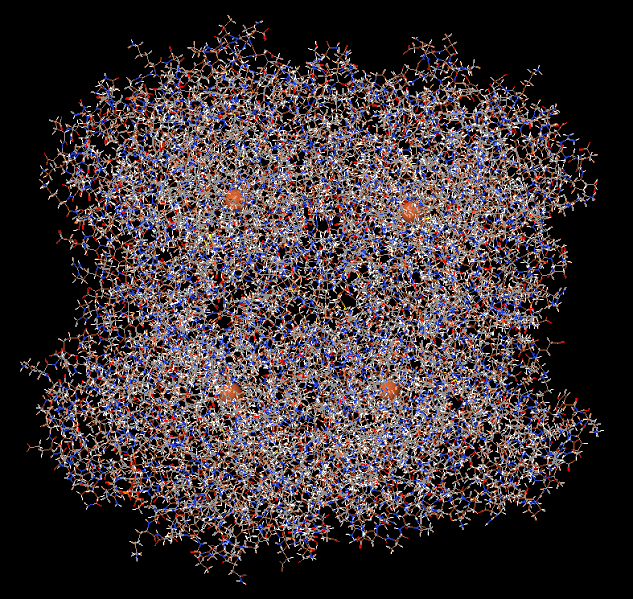
Catalase and four heme groups
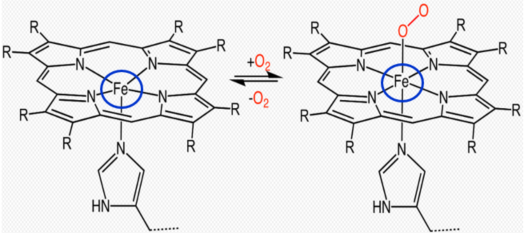
Binding of heme group to oxygen
Catalase Reaction Mechanism
Step 1: Cpd I (compound immediate I ) formation
At the active site of the enzyme, heme donates two electrons to the first molecule of H2O2, one electron from F3+ and the other from the porphyrin ring, forming an intermediate compound called the oxoferryl π-cationic porphyrin radical known as CpdI. As one of the protons, hydrogen ion (H+), of the hydrogen peroxide molecule is removed from one end of the molecule and placed at the other end via a histidine residue in the active site, this process polarizes and breaks the O-O bond in hydrogen peroxide, resulting in the creation of one molecule of water.
Step 2: Oxidation of hydrogen peroxide
The second hydrogen peroxide molecule is oxidized, donating two electrons to CpdI. As a result, Cpd1 returns to its original Fe3+ state, releasing one molecule of water and one molecule of oxygen.
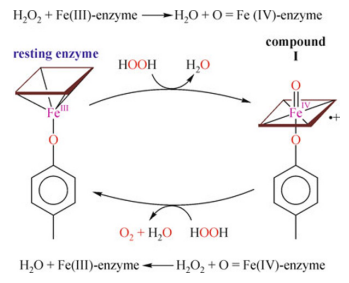
So, to summarize these steps chemically,

We have examined the mechanism by which oxidative stress is reduced through the conversion of reactive oxygen species into harmless water, focusing on the key antioxidant enzymes involved.
[Reference articles]
[1] Perry JJ, Shin DS, Getzoff ED, Tainer JA. The structural biochemistry of the superoxide dismutases.
https://www.ncbi.nlm.nih.gov/pmc/articles/PMC3098211/
[2] Biochemistry, Hexose Monophosphate Pathway
https://www.ncbi.nlm.nih.gov/books/NBK551687/
[3] Glutathione Peroxidase-1 in Health and Disease: From Molecular Mechanisms to Therapeutic Opportunities
https://www.ncbi.nlm.nih.gov/pmc/articles/PMC3159114/
[4] Redox status expressed as GSH:GSSG ratio as a marker for oxidative stress in paediatric tumour patients
https://www.ncbi.nlm.nih.gov/pmc/articles/PMC3506742/
[5] Redox status expressed as GSH:GSSG ratio as a marker for oxidative stress in paediatric tumour patients
https://www.spandidos-publications.com/10.3892/ol.2012.931#b12-ol-04-06-1247
[6] Monofunctional Heme-Catalases
https://www.ncbi.nlm.nih.gov/pmc/articles/PMC9687031/
Human catalase: looking for complete identity
https://link.springer.com/content/pdf/10.1007/s13238-010-0113-z.pdf
Thirty years of heme catalases structural biology