Constant movement of electrons: redox process is the process of life
Oxidation and reduction (Redox) processes, which represent the movement of electrons between molecules within cells, form the basis of biological activity systems. When examined at the atomic level, our existence can fundamentally be described as a sequence of events driven by the movement of electrons. This is because the human body derives energy, sustains cellular functions, and transmits signals through such processes. Therefore, I completely agree with the words of Hungarian Nobel laureate Albert Szent-Györgyi: “Life is nothing but an electron looking for a place to rest.”
“Life is nothing more than an electron looking for a place to rest.”
However, the generation of oxidizing substances (ROS, reactive oxygen species) as a by-product of these processes is unavoidable. When excessive ROS accumulates within cells for various reasons, oxidative stress can occur, which can cause damage to biomolecules. Fortunately, cells are equipped with mechanisms to detect and respond to changes in ROS concentration. By sending signals to these devices, they respond appropriately by rapidly transcribing and expressing genes to cope with and adapt to them. In other words, our human body has its own system to maintain homeostasis by maintaining an appropriate balance between oxidation and antioxidants.
Maintaining cellular homeostasis
For a long time, ROS have been considered toxic by-products of redox reactions, damaging large biomolecules (DNA, RNA, proteins, and lipids) and causing cell death or malignant changes. In addition, it has been the subject of caution as it has been pointed out as a cause of various diseases and aging caused by tissue damage. However, as extensive research has progressed, it has been recognized that ROS are not solely malicious substances that cause damage to the human body. It has been revealed that ROS regulates physiological signaling pathways based on its concentration. For instance, hydrogen peroxide (H2O2), a typical ROS, is maintained within a physiologically appropriate level inside cells through strict control of cellular physiological signaling and antioxidant systems to regulate cell proliferation, differentiation, and death. However, if H2O2 exceeds this optimal concentration range, it can destroy biological macromolecules that regulate the fate of cells. When this optimal range is exceeded, various antioxidant systems are activated to attempt antioxidant reactions and detoxification. At this point, ROS acts as an essential physiological signaling substance. It is also well known that ROS is involved in inflammatory signal transmission in the inflammatory response, which is part of the immune system.
Oxidative eustress
In fact, cells have a mechanism to limit and control the production of H2O2, a representative ROS, and its production location to specific compartments or areas within the cell. In other words, it is possible to ensure that the effect of H2O2 occurs only where it is needed for a specific function within the cell while preventing it from spreading freely to other areas of the cell or the entire cell. Restricting intracellular H2O2 to a specific area can affect various biological processes by selectively activating or inhibiting specific signaling pathways in that area. Because various compartments uniquely react with specific proteins or enzymes that are sensitive to oxidative stress, it enables targeted redox signaling. This local production and action of H2O2 contributes to minimizing potential damage caused by excessive ROS accumulation while maintaining specificity in signaling pathways. Cells promote intracellular homeostasis by maintaining the balance between oxidation and antioxidants through various antioxidant defense systems, including Catalase and other enzymes that remove oxidation. There is also a concept called oxidative eustress, which has a positive meaning, emphasizing that H2O2, adjusted to an appropriate amount, helps smooth physiological activities within cells. This is a concept that runs counter to the concept of oxidative stress, which refers to an undesirable state caused by excessive oxidation.
Let's look at how ROS, such as hydrogen peroxide, act as signaling substances to maintain the balance between oxidation and antioxidation and avoid cell damage when cells experience oxidative stress.
4 transcription factors and Hydrogen peroxide
1. Keap1‐Nrf2 activation pathway
The interaction between Keap1 and Nrf2 (Nuclear factor erythroid 2-related factor 2) represents a critical thiol-based redox switch that responds to diverse oxidative stresses, playing a pivotal role in maintaining redox homeostasis. Keap1 serves as a sensor protein, continuously monitoring the redox status within the cell.
Nrf2 plays an important role in regulating the expression of genes associated with antioxidant defense mechanisms. Under normal conditions, where oxidants such as hydrogen peroxide exist at appropriate levels, Keap1 faithfully acts as an "Nrf2 inhibitory protein" and induces the degradation of Nrf2 in the proteasome, maintaining Nrf2 in a low-activity state and strictly regulating its activity to prevent excessive activation of the Nrf2-mediated signaling pathway. However, during oxidative stress, when the physiological concentration of hydrogen peroxide increases, the cysteine residue in Keap1 undergoes oxidation, forming a disulfide bond. This modification alters the structure of Keap1, leading to the release of bound Nrf2. Once released, Nrf2 translocates from the cytoplasm into the nucleus, where it acts as a master regulator by binding to antioxidant response elements (AREs) present in the promoter regions of target genes involved in antioxidant defense mechanisms, leading to the expression of over 500 antioxidant-related genes. These target genes include various enzymes such as Superoxide dismutase (SOD), Catalase, and Glutathione peroxidase (GPx), which are often referred to as the "three musketeers of reactive oxygen species scavengers." By sensing the concentration of H2O2, Nrf2 restores the homeostasis of intracellular redox balance and helps protect cells from oxidative damage.
☞ Disulfide bonding: A disulfide bond is a covalent bond between two adjacent sulfur(S) atoms that have lost hydrogen through an oxidation process, leading to the transformation of a one-dimensional protein peptide into a more complex three-dimensional structure, such as a tertiary or quaternary structure, necessary for its function. Disulfide bonds play a crucial role in protein structure. Among the 20 amino acids, only two contain sulfur(S): Methionine and Cysteine. In Methionine, the sulfur is positioned in the middle rather than at the end, preventing it from forming disulfide bonds. Therefore, disulfide bonds occur exclusively in Cysteine, which possesses a thiol functional group(-SH). In this process, the thiol groups of two cysteine residues lose all their hydrogen atoms (oxidation) to form a strong covalent bond represented as R−S−S−R′, where R and R' represent different peptide chains or different cysteine residues within the same peptide chain. This chemical bond is also referred to as a disulfide bridge or SS-bond. It contributes to the spatial arrangement of the peptide chain within a protein molecule through folding, providing stability to the protein molecule. The presence of disulfide bonds increases the stability of the protein molecule against external factors. Due to their highly oxidative nature, disulfide bonds can form between or within protein molecules involved in antioxidant defense pathways under oxidative stress conditions, thereby regulating signaling pathways.
The following image demonstrates how the primary polypeptide structure folds into a three-dimensional structure through the formation of disulfide bonds between cysteine(Cys) residues via oxidation. Disulfide bonds play a very important role in the formation of numerous biological proteins, including enzymes and hormones. Despite cysteine comprising only about 2% of the amino acids in vertebrate proteins, over 90% of proteins contain at least one cysteine residue. This highlights the significant role of cysteine and disulfide bonding in maintaining the structural integrity and functionality of proteins essential for various physiological processes in the human body.
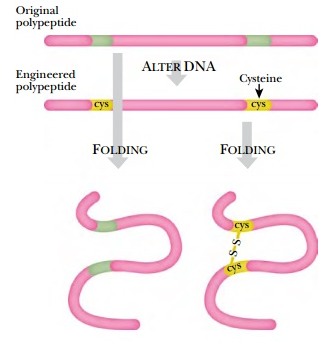
2. FOXO (forkhead box class O) transcription factor
Under non-oxidizing conditions, the FOXO protein is primarily located in the cytoplasm and is degraded by the AKT signaling pathway. However, during oxidative stress when hydrogen peroxide levels are elevated, FOXO forms a disulfide bond with the TNPO protein, facilitating its translocation into the cell nucleus. TNPO serves as a specialized transport system responsible for shuttling FOXO from the cytoplasm to the nuclear membrane. Once in the nucleus, FOXO activates the expression of antioxidant genes. One such example is the upregulation of the Mn-SOD enzyme gene in mitochondria, which utilizes manganese as a cofactor to detoxify superoxide anion radicals by converting them into hydrogen peroxide
3. Hypoxia-inducible factor (HIF)
HIF (Hypoxia-Inducible Factor) is a transcription factor that stimulates and upregulates various genes in response to a hypoxic (low oxygen). The human body generates ATP and maintains redox balance through the electron transport chain(ETC) in the mitochondrial inner membrane. If the electron transport chain(ETC) is not working properly or is inhibited, the production of H2O2 can increase. Hypoxia prevents oxygen from being reduced to water in the final complex within the ETC, causing electrons to react with oxygen molecules(O2) and increasing the amount of H2O2. In a state of high oxidative stress caused by elevated levels of H2O2, HIF moves to the cell nucleus and forms a transcriptional complex by binding to specific DNA sequences called Hypoxia Response Elements(HREs) located in the promoter regions of target genes. This complex then enhances the expression of various genes to cope with hypoxic conditions and promote cellular adaptation. HIF upregulates the expression of genes involved in angiogenesis(major Vascular Endothelial Growth Factor, VEGF), erythropoiesis-stimulating factors like EPO, and glycolytic enzymes for anaerobic metabolism. By increasing anaerobic metabolism and promoting the generation of blood vessels and red blood cells, HIF contributes to an efficient oxygen supply.
4. NF-κB pathway
NF-κB(Nuclear Factor kappa B) is a transcription factor present in almost all cell types and plays a very important role in immune response, inflammation, cell survival, and proliferation. In a physiologically resting state, NF-κB exists in an inactive form in the cytoplasm and is subject to strict regulation by binding to its inhibitor protein, IκB (inhibitors of NF-κB). However, under conditions of stress such as increased inflammatory signals such as cytokines or high levels of H2O2, this inhibitor is degraded, allowing NF-κB to become released and translocate into the cell nucleus. Once in the nucleus, it binds to specific DNA sequences known as κB sites to regulate gene expression.
In this case, hydrogen peroxide(H2O2) as a signaling molecule has the characteristic of acting as both a stimulator and regulator of NF-κB activation, depending on the context. In the cytoplasm, H2O2 facilitates NF-κB release by oxidizing cysteine residues within IκB, the inhibitor of NF-κB, leading to its degradation in the proteasome. In addition, H2O2 can directly affect NF-κB by oxidatively modifying the cysteine residues within NF-κB itself. On the other hand, an increase in H2O2 concentration in the cell nucleus may actually diminish the gene transcription activity of NF-κB by interfering with its DNA binding ability. This modulation can vary based on factors such as cellular context, concentration levels, and duration of exposure.
We have examined several ways in which hydrogen peroxide (H2O2), a prominent ROS, functions as a signaling molecule within cells. Compared to superoxide radicals, H2O2 has higher membrane permeability, making it relatively easier to traverse cellular membranes. Additionally, its stability renders it suitable for signaling functions. While hydroxyl radicals are too reactive to serve as signaling molecules, H2O2's stability and membrane permeability make it one of the most important ROS for cellular signaling. Nonetheless, there are reports suggesting that superoxide radicals can act as growth signals in diverse cells by activating the MAPK/ERK pathway, and singlet oxygen may also mediate various signal transduction pathways and promote programmed cell death. However, due to the limitation in the detection technology of ROS and its nature, many aspects remain ambiguous, and further research into the physiological signaling roles of various ROS is required.
[Reference materials]
Redox signaling at the crossroads of human health and disease
https://www.ncbi.nlm.nih.gov/pmc/articles/PMC8971743/#mco2127-bib-0006
An overview of mechanisms of redox signaling
https://www.ncbi.nlm.nih.gov/pmc/articles/PMC4048798/
Signaling Functions of Reactive Oxygen Species
https://www.ncbi.nlm.nih.gov/pmc/articles/PMC4226395/
Redox Signaling, Vascular Function, and Hypertension
https://www.ncbi.nlm.nih.gov/pmc/articles/PMC2828811/
Reactive oxygen species or reactive sulfur species: why we should consider the latter